The Biomechanics of Muscle Growth
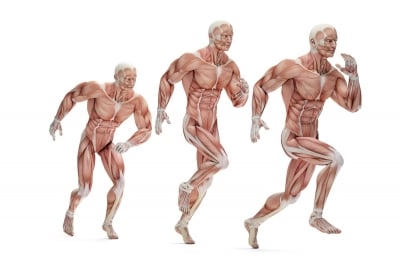
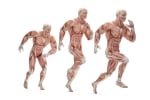
Muscle growth, technically termed as "hypertrophy," is a fascinating process driven by a complex interplay of biomechanical events.
These events, initiated by external stimuli such as resistance training, result in the increase of muscle fiber size.
This article delves deeper into the biomechanics behind muscle growth.
Biomechanics, in its essence, marries the principles of mechanics with the intricacies of biology. It provides a window into understanding how living organisms move, grow, react, and adapt to their surroundings. When examining human performance, athletic prowess, rehabilitation, or even the evolution of species, biomechanics plays a pivotal role. This introduction offers a deeper dive into the world of biomechanics, its origins, relevance in today's world, and its wide-ranging applications.
Historical Perspective
The roots of biomechanics trace back to ancient civilizations, where early scientists and thinkers began observing and documenting human movement. Aristotle, for example, authored "On the Movement of Animals," laying the groundwork for biomechanical thought. Leonardo da Vinci's sketches of human anatomy portrayed his intense interest in the mechanics of the human body. These early endeavors were the foundation upon which modern biomechanics was built.
Defining Biomechanics
Biomechanics can be defined as the study of the structure and function of biological systems using the principles of mechanics. At a more granular level, it examines how forces - both external like gravity and internal like muscle contractions - influence the movement and structure of organisms.
It's segmented into two primary branches:
- Kinematics - Describes the motion of bodies without considering the forces that cause the movement. In human biomechanics, this could refer to the trajectory of a limb during a running stride or the rotation of a joint.
- Kinetics - Focuses on the forces behind those movements, whether it's the muscular forces propelling a sprinter forward or the gravitational force pulling a falling apple towards the Earth.
The Role of Biomechanics in Medicine
One of the most transformative applications of biomechanics is in the field of medicine. Orthopedic surgeons, for example, lean on biomechanical principles when they treat injuries related to movement. By understanding the mechanics of joints, tendons, and bones, they can make informed decisions about treatments, surgical procedures, and rehabilitation.
Rehabilitation professionals, like physiotherapists, use biomechanics to design exercise regimens that restore function and strength without placing undue stress on injured areas. Additionally, the design of prosthetics and orthotics is deeply rooted in biomechanical principles to ensure that these devices replicate, as closely as possible, the natural movement of the body.
Biomechanics in Sports and Fitness
Athletes, coaches, and sports scientists have long been invested in optimizing performance. By analyzing the biomechanics of athletes, they can refine techniques, develop training methodologies, and prevent injuries. For instance, the biomechanics of a baseball pitch can be deconstructed to improve speed and accuracy while minimizing the risk of shoulder or elbow injuries.
Similarly, in the world of fitness, biomechanical analyses can help in crafting exercise routines that are effective and safe. Whether it's perfecting the form of a squat or understanding the optimal stride length in running, biomechanics offers insights that can be transformative.
Biomechanics in Evolutionary Biology
Beyond immediate human applications, biomechanics plays a role in understanding the evolutionary journey of various species. By studying the biomechanics of ancient creatures, scientists can make educated guesses about their behavior, habitat, and survival strategies. The way a long-extinct creature's limb or spine is structured can reveal clues about how it moved, hunted, or escaped predators.
The Modern Age: Technology and Biomechanics
With the advent of technology, the field of biomechanics has undergone revolutionary changes. Tools like motion capture systems, force platforms, and computer modeling allow for precise measurements and analyses. These technologies enable a detailed look at movement, forces, and stresses, which was previously unimaginable.
For instance, gait analysis labs use cameras and sensors to capture the minutiae of an individual's walk or run. Such insights can help in diagnosing abnormalities, refining athletic technique, or even in designing personalized footwear.
Conclusion
Biomechanics is a vast and ever-evolving field, bridging the gap between the physical world and biological organisms. From its historical roots with Aristotle and da Vinci to its modern applications in sports, medicine, and evolutionary biology, biomechanics remains at the forefront of understanding movement and function.
Its relevance today is underscored by the myriad of applications that touch our daily lives. Whether it's improving athletic performance, rehabilitating from injuries, designing ergonomic workspaces, or diving into the mysteries of extinct species, the principles of biomechanics illuminate our understanding and enhance our capacities.
Muscles are more than just the engines that propel our bodies; they are marvels of nature, intricate in design and function. Their architecture is optimized for movement, force production, and adaptability. Understanding the complex structure of muscles provides insight into their ability to respond to mechanical stimuli, a process known as mechanotransduction. Let's delve deeper into these two intertwined facets of muscle biology.
The Hierarchical Structure of Muscle
At a glance, muscles might seem simple, but their structure is hierarchically organized and incredibly complex. Breaking it down:
- Muscle (Organ Level): The whole muscle, such as the bicep brachii, is what we commonly think of when imagining a muscle. It's encased by a sheath called the epimysium.
- Fascicles (Bundle of Fibers): Within each muscle are bundles of fibers called fascicles, which are surrounded by the perimysium.
- Muscle Fibers (Cell Level): Each fascicle contains numerous muscle fibers, or myocytes. These are the actual muscle cells, elongated in structure and ensheathed by the endomysium. Each muscle fiber arises from the fusion of many precursor cells, which explains their multi-nucleated nature.
- Myofibrils: Delving deeper into the muscle fiber, we encounter myofibrils. These are rod-like structures that run parallel to the muscle fiber and occupy most of its volume. It is the myofibrils that contain the machinery for muscle contraction.
- Sarcomeres (Functional Units): Myofibrils are composed of repeating units called sarcomeres, which are the basic functional units of a muscle. Sarcomeres are bordered by Z-lines and contain the proteins actin (thin filaments) and myosin (thick filaments) that slide past each other during muscle contraction.
- Actin and Myosin: These proteins are the primary players in muscle contraction. The myosin heads attach to actin filaments and pull them, causing the sarcomere to shorten and the muscle to contract.
The Marvel of Mechanotransduction
Mechanotransduction is the process through which cells convert mechanical signals into biochemical responses. In the context of muscles, it means that when muscles experience a mechanical stimulus (like lifting weights), they kick-start a series of biochemical pathways that can lead to muscle growth and adaptation.
How does this work at the microscopic level?
- Initial Mechanical Stimulus: When a muscle contracts against resistance, it creates tension within the muscle fibers. This tension is the primary mechanical stimulus.
- Opening of Mechanosensitive Channels: The tension from the stimulus affects the muscle cell membrane (sarcolemma) and its associated structures. This tension can cause certain ion channels, which are sensitive to mechanical stress, to open. These channels allow the flow of calcium ions, among others, initiating an intracellular cascade of events.
- Activation of Signaling Pathways: The entry of ions and the deformation of other cellular structures activate various signaling pathways. For instance, the pathway involving the molecule mTOR (mammalian target of rapamycin) is crucial for muscle protein synthesis and growth.
- Gene Expression and Protein Synthesis: Activated pathways influence the cell's machinery to produce new proteins or ramp up the production of existing ones. This synthesis is fundamental to muscle repair and hypertrophy.
- Muscle Adaptation: Over time, with repeated mechanical stimuli (regular training), the muscle adapts. This can mean increased muscle size (hypertrophy), improved metabolic efficiency, or enhanced resistance to fatigue, depending on the nature and consistency of the stimulus.
The Role of Satellite Cells
Satellite cells, which sit between the sarcolemma and the basal lamina of muscle fibers, play a significant role in the response to mechanical stimuli. When a muscle is damaged or experiences mechanical stress, these cells become activated. They proliferate and then differentiate, fusing to existing muscle fibers. This process contributes new nuclei to the muscle fibers, facilitating the synthesis of more proteins and, thus, muscle growth.
The Importance of Sensitivity
It's crucial to understand that muscles don't always respond to mechanical stimuli in the same way. Their sensitivity to mechanotransduction can be influenced by various factors, including:
- Training Status: A trained individual might have a different response compared to a beginner.
- Age: Younger muscles have a more robust response to mechanical stimuli.
- Nutrition: Adequate protein and calorie intake can modulate the effectiveness of mechanotransduction.
- Hormonal Environment: Hormones like testosterone and growth hormone play roles in the muscle's response to mechanical stress.
Conclusion
Muscles are sophisticated structures, with a hierarchy that facilitates their primary function: contraction. Beyond that, their ability to sense and respond to mechanical stimuli through mechanotransduction is a testament to their dynamic nature. This adaptability ensures that muscles can grow, repair, and evolve in response to the demands placed upon them. Whether you're lifting a heavy weight, rehabilitating an injury, or just going about your daily tasks, remember: every movement is a dance of intricate biomechanics and cellular responses, choreographed to perfection.
Muscles are constantly interacting with their environment, and among the myriad of stimuli they encounter, mechanical tension stands out as one of the most potent. It is a driving force behind muscle adaptation, growth, and strength development. But what exactly is mechanical tension, and why is it so central to muscle physiology? This deep dive will elucidate the pivotal role of mechanical tension in the context of muscle function and adaptation.
Defining Mechanical Tension
Mechanical tension can be understood as the force exerted on a muscle when it's actively contracted against resistance. This force, whether derived from lifting weights, resistance bands, or even one's own body weight, causes a stretch or pull on the muscle fibers, creating tension within them.
It's worth distinguishing between two types of tension:
- Passive Tension: This arises when a muscle is stretched without undergoing active contraction. Think of the tension in your hamstring when you bend forward to touch your toes.
- Active Tension: This is the tension generated during active muscle contraction against a load. For instance, the tension in your biceps as you curl a dumbbell.
While both forms of tension have their roles in muscle physiology, it's the active tension, especially during resistance training, that's particularly influential in muscle adaptation and hypertrophy.
Mechanical Tension and Muscle Fiber Activation
When a muscle contracts against resistance, not all fibers are activated simultaneously. The body recruits muscle fibers based on the magnitude of the load and the type of muscle fiber. Lighter loads primarily engage slow-twitch (Type I) fibers, while heavier loads recruit the fast-twitch (Type II) fibers.
The greater the mechanical tension, the more exhaustive the recruitment becomes. As the tension increases, more and larger motor units (a motor neuron and the muscle fibers it innervates) are activated, ensuring that the muscle generates sufficient force.
Signaling Pathways and Muscle Growth
Mechanical tension is not just a physical force; it's a potent initiator of intracellular signaling pathways. When a muscle experiences tension, it sets off a cascade of molecular events. One of the most critical pathways involves the aforementioned mTOR (mammalian target of rapamycin). Activation of mTOR stimulates protein synthesis, a vital process for muscle repair and growth.
It's a bit like the muscle's way of saying, "This was tough; we need to build more machinery to handle it next time." Over time, with consistent tension from resistance training, this increased protein synthesis can lead to muscle hypertrophy.
Mechanical Tension vs. Muscle Damage
For years, muscle damage (micro-tears in muscle fibers) was believed to be a primary driver of muscle growth. While damage does play a role, recent research suggests that mechanical tension might be a more influential factor.
Muscle damage undoubtedly triggers an inflammatory response, bringing in growth factors and immune cells to repair the tissue. However, it's the mechanical tension that seems to be the primary stimulus for adaptation. In simple terms, while muscle damage contributes to growth, it's the consistent application of mechanical tension that's paramount for long-term hypertrophy.
Duration and Time Under Tension (TUT)
It's not just the magnitude of tension that matters, but also its duration. Time under tension (TUT) is a concept in resistance training that emphasizes the importance of the length of time a muscle remains under stress during a set.
For instance, if one were to lift a heavy weight very quickly for two repetitions, the total TUT would be quite short. Conversely, lifting a moderately heavy weight for 12 repetitions with a controlled tempo would result in a longer TUT. Both scenarios create mechanical tension, but they affect the muscle differently.
Extended TUT, especially with moderate loads, can enhance metabolic stress in the muscle, another stimulus for growth. Therefore, when designing a training regimen, it's essential to consider not only the weight being lifted but also the duration of the tension.
Overloading and Progressive Tension
Muscles are remarkably adaptive. If exposed to the same level of tension repeatedly, they adapt and grow, making that level of tension less challenging over time. This phenomenon is where the principle of progressive overload comes into play.
To continue experiencing muscle growth, there must be a gradual increase in the mechanical tension placed on the muscle. This can be achieved by increasing the weight lifted, adjusting the tempo of repetitions, or modifying other variables like rest periods. By continuously challenging the muscle with increasing tension, one ensures ongoing adaptation and growth.
Tension and Muscle Tone
Apart from growth, consistent application of mechanical tension contributes to improved muscle tone. Even in a relaxed state, muscles that are regularly subjected to tension tend to have a firmer, more defined appearance. This is due to a combination of muscle hypertrophy and neural adaptations that enhance muscle fiber recruitment.
Conclusion
Mechanical tension is a cornerstone in the world of muscle physiology. It's the language muscles understand, signaling them to adapt, grow, and become stronger. From the moment you pick up a weight, to the molecular cascades it triggers, tension is at the heart of it all.
Understanding the role of mechanical tension underscores the importance of resistance training variables, from the weight on the bar to the tempo of the lift. It's not just about moving weight; it's about the tension that weight creates and how it's harnessed for growth and adaptation.
Muscle growth is a fascinating and multifaceted process that's influenced by a variety of stimuli. Among them, muscle damage holds a unique and somewhat paradoxical place. While we often associate damage with negative outcomes, when it comes to muscles, damage can be a precursor to growth and adaptation. Let's delve deeper into the relationship between muscle damage and hypertrophy, the ensuing processes, and its implications for training.
What is Muscle Damage?
When muscles are exposed to unfamiliar or strenuous activity, especially exercises that involve eccentric (lengthening) contractions, they can incur microscopic tears. This is referred to as muscle damage. It's the reason behind the soreness you feel a day or two after a challenging workout, a phenomenon known as Delayed Onset Muscle Soreness (DOMS).
It's crucial to understand that this "damage" isn't necessarily detrimental. In the right context and when allowed proper recovery, it acts as a potent stimulus for muscle growth and adaptation.
Muscle Damage: A Closer Look
- Z-Line Streaming: Within each muscle fiber are sarcomeres, the basic contractile units. The sarcomeres are delimited by Z-lines. During intense muscle activity, particularly with eccentric contractions, these Z-lines can become disrupted, leading to what's known as "Z-line streaming."
- Release of Intramuscular Proteins: Damage can result in the release of proteins like creatine kinase from inside the muscle fibers into the bloodstream. Elevated levels of such enzymes post-exercise can be indicative of muscle damage, though it's worth noting that the relationship between enzyme levels and the extent of damage isn't always linear.
- Inflammation and Immune Response: Once damage is detected, the body initiates an inflammatory response. Immune cells, like neutrophils and macrophages, migrate to the damaged site. While inflammation often gets a bad rap, in this context, it's beneficial. These immune cells clear out debris and release growth factors essential for muscle repair.
Muscle Damage and Growth: The Connection
Muscle damage acts as a potent stimulus for muscle repair and growth. Here's how:
- Satellite Cell Activation: As mentioned earlier, satellite cells lie dormant between the muscle fiber and its surrounding sheath. Upon muscle damage, these cells become activated. They proliferate and fuse with the damaged muscle fiber, donating their nuclei. More nuclei mean a greater capacity for protein synthesis and, consequently, muscle growth.
- Upregulation of Protein Synthesis: Muscle damage activates various intracellular signaling pathways, including the mTOR pathway. This upregulation boosts muscle protein synthesis, the process by which new proteins are constructed, leading to muscle repair and hypertrophy.
- Remodeling of Muscle Architecture: Chronic exposure to damaging stimuli can lead to the remodeling of muscle fibers. This doesn't just mean an increase in size, but also changes in the muscle's architecture, making it more resistant to future damage—a phenomenon called the "repeated bout effect."
The Balance: Damage vs. Recovery
While muscle damage can stimulate growth, it's only part of the equation. Recovery is equally, if not more, essential. Without adequate recovery, the muscle doesn't have the opportunity to repair and grow efficiently.
- Nutrition: Post-exercise, muscles are like sponges, eager to absorb nutrients. Consuming protein, with its muscle-building amino acids, alongside carbohydrates, helps jumpstart the recovery and growth process.
- Rest: It's during rest that the magic happens. Muscle protein synthesis peaks and the body actively repairs the damage. Skipping this crucial phase can lead to overtraining, where damage accumulates faster than the body can repair, hindering growth and increasing injury risk.
- Active Recovery: Gentle movement, like walking or light stretching, can enhance blood flow to sore muscles, aiding in the removal of waste products and delivering nutrients more efficiently.
Training Implications
Understanding muscle damage and its role in growth can inform training strategies:
- Variety: Incorporating new exercises or altering the tempo can introduce unfamiliar stimuli to the muscles, eliciting damage and promoting growth.
- Eccentric Emphasis: Since eccentric contractions are particularly effective at inducing muscle damage, exercises that emphasize this phase, like slow negatives, can be potent growth stimulators.
- Volume and Frequency: While muscle damage is beneficial, it's easy to overdo it. It's essential to find a balance in training volume and frequency, ensuring muscles have adequate time to recover before being subjected to another damaging stimulus.
- Listen to Your Body: While some soreness post-exercise is expected, excessive or prolonged soreness might indicate too much damage and insufficient recovery. Adjusting training variables in such cases is crucial.
Conclusion
Muscle damage, while sounding ominous, is a natural and integral part of the muscle growth process. It's a testament to the body's remarkable ability to adapt and improve. When a muscle is damaged, it doesn't just aim to repair back to its original state—it aims to become better, stronger, and more resilient. As with many things in life, it's about balance. The interplay between stimulus (damage) and response (recovery and growth) underscores the importance of a well-rounded training and recovery regimen for optimal muscle hypertrophy.
When discussing the mechanisms that drive muscle growth, metabolic stress often emerges as a principal factor alongside mechanical tension and muscle damage. Although it doesn't involve the same physical disruptions as muscle damage, metabolic stress can induce potent physiological responses that contribute to muscle hypertrophy. Let's delve into the intricate world of metabolic stress and its influence on muscle growth.
What is Metabolic Stress?
Metabolic stress refers to the physiological processes that occur in the muscle due to the accumulation of byproducts from metabolism during intense exercise. When muscles contract repeatedly, as they do during resistance training, they rely on stored energy substrates. This process results in the production of various metabolites, including lactate, hydrogen ions, and inorganic phosphate. The accumulation of these metabolites, in part, contributes to the 'burning' sensation you feel during prolonged sets.
Causes of Metabolic Stress
- Anaerobic Glycolysis: During high-intensity exercises, muscles predominantly utilize anaerobic glycolysis for energy, leading to the production of lactate. As lactate accumulates, it increases the acidity inside the muscle, contributing to metabolic stress.
- Adenosine Triphosphate (ATP) Degradation: ATP is the primary energy currency of cells. During exercise, ATP is broken down to release energy, leading to an accumulation of adenosine diphosphate (ADP) and inorganic phosphate. Excessive inorganic phosphate can impair muscle contraction and contribute to fatigue.
- Oxygen Deprivation: Intense muscle contractions can temporarily compress and reduce blood flow to the working muscles. This transient hypoxia or oxygen deprivation can further enhance metabolic stress.
Metabolic Stress and Muscle Hypertrophy: The Connection
Metabolic stress plays a multifaceted role in promoting muscle growth:
- Cellular Swelling: The accumulation of metabolites draws water into the muscle cells, causing them to swell. This cellular swelling can exert a stretch on the cell membrane, potentially acting as a mechanical signal for muscle growth.
- Hormone Production: Metabolic stress can stimulate the release of anabolic hormones, including growth hormone and insulin-like growth factor 1 (IGF-1). These hormones can promote protein synthesis and inhibit protein breakdown, creating an environment conducive to muscle growth.
- Increase in Muscle Fiber Recruitment: To counteract the effects of accumulating metabolites and maintain force production, the body can recruit additional motor units. This increased recruitment can stimulate a broader range of muscle fibers, promoting overall muscle hypertrophy.
- Activation of Satellite Cells: Metabolic stress can also activate satellite cells, dormant cells that play a crucial role in muscle repair and growth. Once activated, these cells can fuse to existing muscle fibers, donating additional nuclei and boosting the muscle's capacity for protein synthesis.
- Enhancement of Muscle Protein Synthesis: Metabolic stress has been shown to upregulate muscle protein synthesis, a foundational process for muscle repair and hypertrophy.
Practical Implications: Training for Metabolic Stress
Training specifically to induce metabolic stress often involves techniques that maximize metabolite accumulation:
- High Repetition Sets: Performing exercises with a high number of repetitions (15-30 or more) using moderate to light weights can enhance metabolic stress. The prolonged set duration allows for significant metabolite accumulation.
- Short Rest Intervals: Shortening the rest period between sets (e.g., 30 seconds to a minute) limits the clearance of metabolites, compounding the metabolic stress in subsequent sets.
- Advanced Techniques: Techniques such as drop sets, supersets, or giant sets can prolong muscle tension and increase the time under metabolic stress.
- Blood Flow Restriction (BFR) Training: BFR involves restricting venous blood flow return (but not arterial inflow) using wraps or cuffs. This technique, when combined with low-load resistance training, can amplify metabolic stress and has been shown to stimulate muscle growth.
Balancing Metabolic Stress with Other Factors
While metabolic stress is a potent stimulus for muscle growth, it's essential to balance it with other growth-inducing factors:
- Avoid Overreliance: While training for metabolic stress can be effective, over-relying on it at the expense of heavy, tension-focused training can limit overall muscle development. A comprehensive training program should integrate various stimuli, including mechanical tension and muscle damage.
- Nutrition and Recovery: Metabolic stress, like other forms of training stimuli, necessitates recovery. Consuming a post-workout meal rich in protein and carbohydrates can aid in replenishing glycogen stores, mitigating muscle protein breakdown, and enhancing muscle protein synthesis.
- Monitor Fatigue: Training sessions that emphasize metabolic stress can be fatiguing. It's essential to monitor signs of overtraining, such as prolonged soreness, reduced performance, or disturbed sleep, and adjust training variables accordingly.
Conclusion
Metabolic stress stands as a testament to the body's intricate ways of adapting to challenges. What may seem like a mere burning sensation in the muscles is, in fact, a cascade of processes promoting growth and adaptation. By understanding metabolic stress and strategically incorporating it into a training regimen, one can harness its benefits for enhanced muscle hypertrophy, alongside other crucial growth stimuli.
While the biomechanics of muscle growth are driven primarily by resistance training and its various associated factors, the role of nutrition in this process is unequivocally paramount. Nutrition provides the raw materials required for muscle repair, recovery, and growth. Let's explore the intimate relationship between nutrition and muscle hypertrophy and how dietary choices can influence muscle-building outcomes.
The Foundation: Energy Balance
Muscle growth requires energy. At the most basic level, one needs to consume more calories than they expend – a state known as a caloric surplus.
- Caloric Surplus: Creating a moderate caloric surplus provides the extra energy necessary for muscle protein synthesis. However, the size of the surplus matters. Excessive caloric intake can lead to disproportionate fat gain.
- Caloric Deficit and Muscle Growth: It's more challenging to build muscle in a caloric deficit. However, with appropriate protein intake and resistance training, muscle maintenance or even modest growth is possible, especially for beginners or those returning after a break.
Macronutrients: The Building Blocks
- Protein: Arguably the most discussed macronutrient in the context of muscle growth.
Amino Acids: Proteins are composed of amino acids, often referred to as the building blocks of the body. Of these, the branched-chain amino acids (BCAAs) - especially leucine - play a pivotal role in muscle protein synthesis.
Optimal Intake: Recommendations vary, but consuming 1.2 to 2.2 grams of protein per kilogram of body weight daily is often suggested for those engaged in resistance training. It's beneficial to distribute this intake across multiple meals to maximize muscle protein synthesis throughout the day. - Carbohydrates:
Energy Source: Carbohydrates provide the primary energy source for high-intensity workouts. They replenish glycogen stores, ensuring muscles have the necessary fuel for subsequent workouts.
Post-Workout Nutrition: Consuming carbohydrates post-workout can enhance glycogen repletion and may expedite muscle recovery. - Fats:
Essential Roles: Fats play roles in hormone production, including testosterone and estrogen, which can influence muscle growth. Additionally, they support overall health and the absorption of fat-soluble vitamins.
Balanced Intake: While fats are calorie-dense, providing 9 calories per gram, they should be consumed in moderation, focusing on sources rich in omega-3s and monounsaturated fats.
Micronutrients and Other Factors
- Vitamins and Minerals: Essential for a plethora of bodily functions, certain vitamins and minerals like Vitamin D, calcium, and zinc play roles in muscle function, bone health, and hormone regulation.
- Hydration: Water is a crucial component of muscle cells. Staying hydrated supports optimal muscle function, aids recovery, and can enhance exercise performance.
- Timing: The concept of nutrient timing—consuming specific nutrients at strategic times, like the post-workout "anabolic window"—has been debated. While nutrient timing can be beneficial, especially for elite athletes, the total daily intake is more crucial for the average individual.
Supplements: Do You Need Them?
Given the vast array of muscle-building supplements available, it's essential to discern which, if any, can be beneficial.
- Creatine: One of the most researched supplements, creatine has been shown to increase muscle mass, strength, and exercise performance. It's safe for most people and can be a valuable addition to a muscle-building regimen.
- BCAAs: Branched-chain amino acids, especially leucine, can stimulate muscle protein synthesis. While they can be beneficial, those consuming adequate protein from whole foods likely don't need an additional BCAA supplement.
- Protein Powders: Whey, casein, soy, and pea are among the various protein powders available. They can be convenient sources of protein, especially for those who struggle to meet their daily intake through food alone.
- Others: Many other supplements claim to boost muscle growth, including beta-alanine, beta-hydroxy beta-methylbutyrate (HMB), and L-carnitine. While some have supportive research, it's essential to consult with a healthcare professional before starting any supplementation.
Dietary Strategies for Muscle Growth
- Cycling Calories: Some athletes and bodybuilders practice caloric cycling, where they alternate between periods of caloric surplus (for muscle growth) and caloric deficit (to reduce fat). This approach aims to maximize muscle gain while minimizing fat accumulation.
- Intermittent Fasting (IF): While IF focuses primarily on eating windows, it doesn't dictate caloric or macronutrient intake. It can be adapted for muscle growth by ensuring a caloric surplus and adequate protein intake within the eating window.
Conclusion
Nutrition and muscle growth are inextricably linked. While resistance training provides the stimulus, nutrition offers the materials and tools required for muscle repair and hypertrophy. By understanding the vital role of nutrition and making informed dietary choices, individuals can optimize their muscle-building potential, ensuring that their hard work in the gym translates into tangible, muscle-bound results.
When discussing the formula for muscle growth, the spotlight often shines brightest on intense training and meticulous nutrition. However, an often-underestimated component that plays an equally vital role is rest and recovery. The body's adaptive processes, responsible for muscle growth and improved performance, predominantly occur during rest periods. Let's delve deeper into why rest and recovery are indispensable in the muscle-building equation.
Muscle Recovery: More Than Just Downtime
When you engage in resistance training, you're essentially creating micro-tears in the muscle fibers. While this might sound alarming, it's a completely natural process and a key driver for muscle hypertrophy. The magic happens during the recovery phase, where these tiny tears are repaired and reinforced, leading to muscle growth. Without adequate recovery:
- Stunted Muscle Growth: The repair and build phase is curtailed, limiting hypertrophy potential.
- Increased Risk of Injury: Overworked muscles and joints can become susceptible to strains, sprains, and more severe injuries.
- Chronic Fatigue: Over time, the cumulative effect of inadequate rest can lead to overtraining syndrome, characterized by prolonged fatigue, decreased performance, and even mood disturbances.
Sleep: The Ultimate Recovery Tool
Sleep is when the body undergoes its most profound recovery and repair processes:
- Hormonal Regulation: Growth hormone, vital for muscle growth, is primarily released during deep sleep. Prolonged sleep deprivation can lead to imbalances in other hormones like cortisol, a stress hormone, further impeding recovery.
- Enhanced Protein Synthesis: The body's ability to repair and build muscle proteins is elevated during sleep.
- Cognitive and Emotional Recovery: Beyond the physical realm, sleep is crucial for cognitive function and emotional well-being. A well-rested mind can ensure motivation, focus, and mental stamina during workouts.
Active Recovery: Beneficial Movement
While rest often connotes complete inactivity, there's merit in including light, non-strenuous activities on "off" days, termed as active recovery. Activities like walking, cycling at a relaxed pace, or even gentle yoga can:
- Boost Blood Circulation: Enhanced circulation can facilitate nutrient delivery to muscles, potentially speeding up recovery.
- Mitigate Stiffness: Gentle movement can reduce muscle tightness and improve flexibility.
- Mental Refreshment: Active recovery can serve as a mental break from intense training routines, helping prevent burnout.
Recovery Strategies: Beyond Sleep and Rest
- Hydration and Nutrition: Drinking adequate water supports metabolic processes vital for muscle repair. Post-workout nutrition, especially protein and carbohydrates, can kickstart recovery.
- Stretching: Regular stretching can enhance muscle flexibility, reduce tightness, and potentially accelerate muscle recovery.
- Massage and Foam Rolling: These modalities can alleviate muscle tightness, improve blood flow, and reduce delayed onset muscle soreness (DOMS).
- Cold and Heat Therapies: Cold showers or ice baths can reduce muscle inflammation, while heat therapy, like saunas or warm baths, can relax tight muscles.
- Compression Garments: Wearing compression clothing post-workout can enhance blood circulation, potentially aiding in faster recovery.
Listening to Your Body: The Ultimate Gauge
The optimal recovery time can vary based on the individual, the intensity of the workout, and other factors. While general guidelines are useful, it's crucial to attune oneself to the body's signals. Persistent soreness, fatigue, irritability, and sleep disturbances can all be indicators that more recovery time or modified training is needed.
Conclusion
The biomechanics of muscle growth, as revealed through our exploration, is not a mere consequence of lifting weights. It is a symphony of interconnected physiological processes, each playing a pivotal role in determining the magnitude and quality of muscle development. Understanding these intricacies provides a nuanced perspective on the journey to muscular hypertrophy, revealing it as more art than mere science.
Tension lays the foundation, creating a mechanical environment where muscles are challenged, forcing them to adapt and grow. The very act of resisting weight, of pushing against a force, signals the body's intrinsic need to prepare for similar future demands, thereby facilitating muscle growth.
Damage, often perceived negatively, is a natural response to resistance training. It's a signal for repair and fortification, a call-to-arms for the body's regenerative processes to heal and enhance muscle fibers, making them more robust than before.
Metabolic stress adds another layer to this complex tapestry. The build-up of metabolites and the subsequent cellular environment it creates, while uncomfortable, pushes the muscle cells to adapt, grow, and become more efficient.
Nutrition, the sustenance of all life processes, provides the essential raw materials. The amino acids from proteins repair the damage, carbohydrates replenish energy stores, and fats ensure hormonal balance. Micronutrients and hydration further enhance the body's ability to optimize muscle growth, revealing that what we consume is just as vital as how we train.
Lastly, rest and recovery underline the importance of pause in a world that often glorifies constant action. It's a humbling reminder that growth, in its truest sense, often happens in stillness, in moments of respite and reflection.
The intricate dance between these factors underlines a profound truth: achieving optimal muscle growth is not about extremes, but balance. It's about understanding the nuanced interplay between exertion and relaxation, intake and metabolism, challenge and recovery.
In wrapping up our exploration, it becomes evident that the pursuit of muscle growth is not just a physical endeavor but a holistic one. It invites individuals to be more in tune with their bodies, to respect their limits, and to appreciate the remarkable adaptability of the human form. By embracing the principles of biomechanics, one not only optimizes muscle growth but also fosters a deeper connection with their own body, leading to a journey of not just physical, but also personal growth.